Characteristics of the Lead-Acid Battery
Mechanical Structure
The basic lead-acid battery has changed little in concept since the 1880's
although improvements in materials and manufacturing methods continue to
bring improvements in energy density, lifetime, and reliability.
A battery consists of a combination of electro-chemical primitive cells.
Cells are the building blocks of which batteries are constructed. A primitive
cell normally consists of the following principal components:
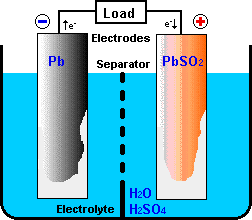 |
- a positive electrode (anode) that receives electrons from the external
circuit when the cell is discharged,
- a negative electrode (cathode) that donates electrons to the external circuit
as the cell discharges,
- an electrolyte which provides a mechanism for charge to flow between positive
and negative electrodes, and
- a separator or spacer which electrically isolates the positive and negative electrodes.
In some designs, physical distance between the electrodes provides the
electrical isolation and the separator is not needed.
Since such a primitive cell will generate a voltage of about 2V, a battery
will be assembled from a series connection of different primitive cells
to obtain the required battery voltage.
For commercial batteries, the components of the primitive cells and
the cell connectors will be mounted and packed in a solid package for proper
and save handling.
|
The mechanical structure of lead-acid batteries consist of flat pasted
plates (the electrodes) immersed in a pool of a sulphuric acid and water
solution (the electrolyte).
The plates are packed together in a comb-like structure.
Two such plate sets (a positive and a negative) from the basic cell block
of the battery.
Such a basic cell block thus consisting of a parallel connection of
primitive cells.
The plates itself consist of a metal grid (current collector) used for
the electrical connection, pasted with lead oxide (PbO2
for the positive plates or lead (Pb) for the negative plates. This lead
coverage is the chemical active layer of the cell. In order to increase
this active surface, the lead and lead oxide coverage is given a porous
structure.
Plate thickness (of the positive plate) is related to lifetime of the
battery because of a factor called "positive grid corrosion". Over time,
the positive plate gets gradually eaten away, so eventually the complete
material is consumed. The corroded material is deposed as sediment at the
bottom of the battery. Thicker plates are directly related to longer life.
Staring batteries typically have plates about 1mm, while deep-cycle batteries
may have plates of about 6mm thick.
A complete battery consists of a series connection of a number of basic
cell blocks. A 12V battery will contain 6 basic cell blocks.
The electrolyte is a sulphuric acid - water solution (typically 30% acid
-70% water at full charge). During the lifetime of the battery, and especially
during recharging some water may evaporate from the electrolyte. Therefore
regular water addition is required for most forms of wet-cell batteries.
Newer low-maintenance types come with excess electrolyte that is calculated
to compensate for water loss during a normal lifetime.
Chemistry of the Lead-Acid Battery
|
The heart of the lead-acid battery is a reversible electro-chemical reaction,
that can produce a voltage difference between the involved metal electrodes.
The basic construction has been shown before;
the lead-acid battery is made up of plates (electrodes):
the negative electrode (cathode) is covered
with lead (Pb)
and the positive electrode (anode) is covered with lead oxide (PbO2).
Both electrodes are immersed in a 35% sulphuric acid and 65% water solution
(H2SO4 + H2O).
In the electrolyte the sulphur-oxide crystals H2SO4
are split into free H+ and HSO4- ions.
|
Discharging
During the discharge process, the positive electrode receives electrons
from the external circuit.
These electrons will react with the active materials of the positive
electrode in a "reduction" reactions that continue the flow of charge
through the electrolyte to the negative electrode.
In this reduction reaction,
PbO2 of the active material will be converted to
PbSO4 absorbing HSO4- and H+
from the electrolyte and electrons from the external load.
As a by-product water is produced.
At the negative electrode, an "oxidation" reactions between the active
materials of the negative electrode and the charge flowing through the
electrolyte results in surplus electrons that can be donated to the external
load closing the electrical loop.
In this oxidation reaction, the Pb active material is oxidized to
PbSO4 absorbing HSO4-
from the electrolyte and giving H+ ions to the electrolyte while
loading electrons to the negative electrode.
Remember that the system is electrically closed: for every
electron generated in an oxidation reaction at the negative electrode,
there is an electron absorbed in a reduction reaction at the
positive electrode.
At the same time H+ ions are generated in the electrolyte at the
negative electrode and absorbed at the positive electrode.
So for the external electron flow through the load, there is an
internal opposite H+ flow through the electrolyte.
The electrolyte is always a part of the electrical circuit!
As the discharge process continues, the electrolyte loses sulphur and
the active materials become depleted absorbing this sulphur as as PbSO4.
As the sulphur concentration is the electrolyte decreases, the chemical
reactions slow down until finally, the battery is no longer capable of
supplying electrons to the external load.
At this point the electrolyte is primary water and the battery is
discharged.
Both electrodes are now covered with PbSO4.
This PbSO4 tends to crystallize, building a hard
crystalline coating, which is hard to break down and this may
destroy the electrodes.
Therefore discharged batteries should be recharged as soon as possible
to avoid this sulphation of the electrodes.
If the battery is recharged, the sulphur is returned to the electrolyte
and the electrodes are chemically converted back into lead oxide (anode)
and lead (cathode).
Recharging
The chemical reactions in the lead-acid cell are reversible. By reversing
the flow of electrons i.e. by putting electrical current in rather than
taking it out, the chemical reactions are reversed to restore active material
that had been depleted.
Chemical Reactions
The scheme below shows the detailed chemical electrode reactions:
Discharge |
Charge |
Positive Electrode
Anode (+) |
Negative Electrode
Cathode (-) |
Positive Electrode
Anode (+) |
Negative Electrode
Cathode (-) |
PbO2 + HSO4-
+ 3H+ + 2e-
PbSO4 + 2H2O |
Pb + HSO4-
PbSO4 + H+ + 2e- |
PbSO4 + 2H2O
PbO2 + HSO4- +
3H+ + 2e- |
PbSO4 + H+ + 2e-
Pb + HSO4- |
Overall Cell Reaction |
Overall Cell Reaction |
Pb + PbO2 +
2H+ + 2HSO4-
2PbSO4 + 2H2O |
2PbSO4 + 2H2O
Pb + PbO2 + 2H+ + 2HSO4- |
Electrical Characteristics
A battery is an accumulator for electric energy: it can be "loaded" (charged)
with energy when e.g. the engine is running (alternator) and can later
release the energy previously stored in it's chemical contents.
The efficiency of this charge-discharge cycle depends on the quality
of he electrolyte-electrode system of the battery and on the efficiency
of the charger system.
Two parameters are commonly used to describe battery performance: voltage
and capacity.
In simple terms, the voltage is the force driving each of the electrons
coming out of a battery and the capacity is the number of electrons that
can be obtained from a battery.
The voltage of a battery cell is determined by the chemical
materials used in it.
The reduction and oxidation reactions mentioned above, each produce
a fixed potential.
The sum of the reduction and oxidation potentials determines
the voltage of the cell.
The discharge reaction at the positive electrode for a lead-acid cell
has a "potential" of 1.685 Volt. The reaction at the negative electrode
has a potential of 0.356 Volt. So the overall voltage of a lead-acid cell
is 2.04 Volt. This value is known as the standard electrode potential.
Other factors, such as the acid concentration can also effect the voltage
of a lead-acid cell. The typical open circuit voltage of commercial lead-acid
cells is around 2.15 Volt.
The voltage of any battery cell is determined by the cell chemistry.
Using other chemical materials different cell voltages may be obtained.
Whereas lead-acid cells have about 2.0 Volt, Nickel-cadmium cells have
a cell voltage of about 1.2 Volt, and the cell voltage of lithium cells
may be as high as 4 Volt.
Moreover, cells can be connected together in series (cascaded) so that
their cell voltages can be accumulated.
This way lead-acid batteries with nominal voltages of 2V, 4V, 6V, etc.
can be assembled.
While the voltage of a cell is fixed by its chemistry, cell capacity
is variable depending on the quantity of active materials it contains.
Capacity is given as the Ampere-Hour product.
The most common definition of capacity is the Ampere-Hour product obtained
if the battery is discharged at an ambient temperature of 25°C with a
constant load current over a time span of 20 hours without the cell voltage
dropping below 1.75V. The capacity value obtained in this way (C-20) is called
"nominal capacity". The actual amount of energy that can be recovered from a
battery substantially depends on the ambient temperature, on the load with
which the battery is discharged and on the age of the battery.
Individual cells may range in capacity from fractions of an ampere-hour
to many thousands of ampere-hours.
A typical capacity value for a starting battery is 75Ah.
Batteries are assembled connecting cells electrically together to obtain
the required voltage and capacity. Connecting cells together in series
will increase the battery voltage, whereas connecting cells (of equal voltage)
in parallel will increase the capacity of the resulting battery.
With a suited combination of parallel and serial cell connections almost any
specific battery voltage and battery capacity can be obtained.
Cells used for battery assemblies should always be identical.
Mixing cells of different chemistry or different size may be
hazardous and should be avoided.
In operation the remaining capacity of a battery is commonly expressed
in percentage of the nominal capacity and referred to as
"State-of-Charge" (SoC).
For a specific type of battery, the State-of-Charge, the electrolyte
concentration and the cell Voltage are closely related.
As the battery is discharged the concentration of sulphur in the
electrolyte decreases, resulting in a decrease of specific weight.
Some batteries allow access to the electrolyte for maintenance.
For such batteries the specific weight (density) of the electrolyte
can be measured.
The following table summarizes the relation between "State of Charge",
Specific Weight of the electrolyte and Battery Voltage for a 12V lead-acid
battery system:
State of Charge
(SoC) |
Electrolyte
[kg/l] |
Voltage
[V] |
Comments |
100% |
1.265 |
12.65 |
The values are valid for an electrolyte temperature of 25°C.
At 10°C the correction for the specific weight is -0.012 kg/l
and -0.072 V for the cell voltage.
The same positive corrections are valid at 40°C.
Measured voltage values are reliable only if the battery has been resting
for at least 4 hours.
|
|
75% |
1.225 |
12.45 |
50% |
1.190 |
12.24 |
25% |
1.155 |
12.06 |
0% |
1.120 |
11.89 |
Notice:
Sulphation of batteries starts when the specific weight of the electrolyte
falls below 1.225 kg/l or when the voltage measures less than
12.5V. Sulphation hardens the battery plates, reducing and eventually destroying
the ability of the battery to store electric energy.
Practically this means that if durability of a lead-acid battery system
is an issue:
only about 25% of the nominal capacity of the
system should be used (up to 50% for deep-cycle battery types),
whenever possible, the battery system should be kept
in a fully charged state.
In a fully charged battery the electrolyte consists of about 35% sulphuric acid
(H2SO4 + H2O)
and 65% water. This mixture will freeze at about -60°C.
In a discharged state, the sulphuric acid is largely absorbed in the battery plates
and the electrolyte is basically water. In this state the electrolyte will
freeze at about -20°C.
If the electrolyte is frozen it looses conductivity resulting in a complete
- and usually permanent - battery failure.
Energy Density
Although the active mass of a lead-acid battery typically has an energy density
of about 160Wh/kg, the energy density of practical assemblies are only in
the range of 25 to 45 Wh/kg.
This is due to limitations in active mass utilization (40% to 60%) and the
weight of electro-chemical and mechanical accessories needed to encapsulate
the aggressive chemicals of the electrolyte and to make the battery practical
and save to handle.
For 12V lead-acid batteries the energy density typically
will be in the range of 3.5 Ah/kg.
But keep in mind that these are nominal values and that in order to prevent
systematic sulphation of the battery system this value will be only about
1 Ah/kg in practical applications.
Temperature Effects on Batteries
Battery capacity strongly depends on the battery temperature:
capacity is reduced as temperature goes down and increases
as temperature goes up.
If the nominal rating is 100% at +25°C (room temperature),
then the capacity is reduced to around 50% for a battery temperature of
-25°C whereas at +50°C the capacity increases to about 120%.
In contrast to battery capacity, the battery lifespan increases with lower
temperatures. At an average working temperature of -25°C battery capacity
is reduced to 50% compared to a temperature of +25°C, but battery life will
increase to about 160% of the lifespan at room temperature. This is providing
the same cycle depth is used, because battery life is also directly related
to how deep the battery is cycled. If a 50% cycle depth is taken as reference,
reducing the cycle depth to 25% will double the lifespan, whereas increasing
the cycle depth to 80% will half the battery's lifespan.
But is does not make much sense using large battery systems to limit the
cycle depth to less that 10%. This is because at very shallow cycles,
the Lead-DiOxide tends to build up in clots on the positive plates rather
than as a thin film, leading to local sulphation.
Also the battery charging voltages (absorption voltage and float voltage)
change with temperature.
For AGM batteries the absorption voltage will vary from about 2.74 volts
per cell (16.4 V for a 12 V battery) at -40°C to 2.30 volts per cell
(13.8V for a 12V battery) at +50°C. This is why a good battery charger
will have a temperature compensation, with a temperature sensor thermally
connected to the battery.
Finally, the self-discharge rate of lead-acid batteries strongly depends
on temperature. This is especially an issue when a battery is stored or
left at rest for a while. An increase of 15°C storage temperature will
approximately double the self-discharge rate. Therefore, unused batteries
should be kept dry and cool.
|